The novel coronavirus is holding up a mirror for our species, giving us an opportunity to consider our place in the evolution of life on Earth and question our anthropocentrism. What I’ve missed during this pandemic and shutdown of our usual social and economic life is writing or reporting that puts this experience, unprecedented in our lifetimes, in context. I haven’t seen news reports, articles or essays that give the “big picture.” For me, what I want and need is an ecological and philosophical context to help me understand coronavirus. The pundits and op-ed writers focus on the human-interest personal stories, the economic and social disruptions, the lockdowns, layoffs, overwhelmed hospitals, lonely deaths. But what does this mean, in the big picture of life? What can we learn, how can we change? I needed to learn a lot more and write down my thoughts—writing is my way of thinking through things for myself. That’s what I’ve done here.
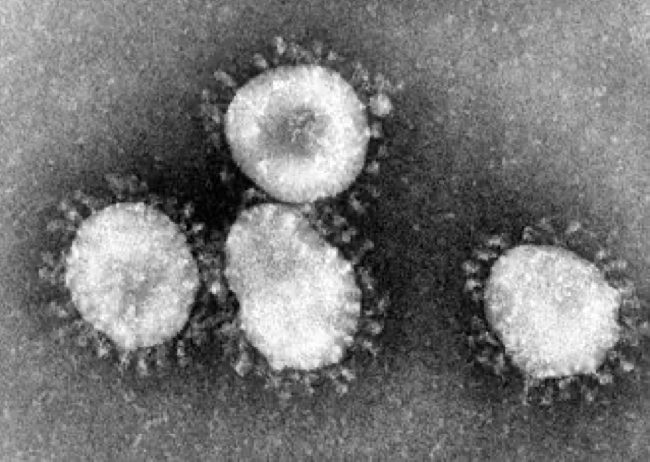
Before we plunge into all that, indulge me to set the stage with a favorite anecdote. When J.B.S. Haldane, a British evolutionary geneticist of the last century, was asked by a group of theologians if anything could be concluded about God from the study of natural history, he is said to have replied “he has an inordinate fondness for beetles.” Haldane was referring to the fact that the taxonomic order Coleoptera, the beetles, account for about 25 percent of all animal species, and around 40 percent of all insects. There are around 400,000 species of beetles, compared to only about 6,400 species of mammals (one of which is ours truly). Haldane was being a bit of a wise guy, as was his wont, but challenging a shallow, anthropocentric view of life.

In his remark about beetles, Haldane was comparing evolutionary diversity only in the so-called higher organisms—the multicellular organisms that belong to the major domain of living things called the eukaryotes. Eukaryotes include all animals, plants, and fungi, which make up pretty much all the living creatures we can see with our naked eyes. Around 1.9 million such multicellular species have been described and scientifically named, out of the 10 million that are thought to currently exist.
But ah, what worlds are hidden from us at smaller scales, out of sight! And because out of sight, often out of mind; we are in a sense handicapped by our large size. In 1676, a Dutchman named Antoni van Leeuwenhoek, peering through a microscope he had constructed, noticed some strange little blobs and published his observations about them. They were bacteria, and van Leeuwenhoek had opened our eyes to the wondrous world of microbiology. It took about two centuries before it became clear that some of these microscopic critters were the cause of a number of infectious human diseases—bubonic plague, tuberculosis, cholera, syphilis, and typhoid fever—and the “germ theory” of disease was born.

Stephen Jay Gould, the noted Harvard paleontologist and evolutionary biologist, called us on our biological scale bias, as Haldane had for our anthropocentrism. In a 1996 essay titled “Planet of Bacteria,” Gould commented on Haldane’s statement about God’s fondness for beetles:
“Fair enough, if we wish to honor multicellular creatures, but we are still not free of the parochialism of our scale. If we must characterize a whole by a representative part, we certainly should honor life’s constant mode. We live now in the ‘Age of Bacteria.’ Our planet has always been in the ‘Age of Bacteria,’ ever since the first fossils—bacteria, of course—were entombed in rocks more than 3 billion years ago. On any possible, reasonable or fair criterion, bacteria are—and always have been—the dominant forms of life on Earth. Our failure to grasp this most evident of biological facts arises in part from the blindness of our arrogance but also, in large measure, as an effect of scale.”
Hmmm… so the creator seems to have an inordinate fondness for bacteria, even more than for beetles! And how many species of such microscopic beings might there be? A 2016 study funded by the National Science Foundation estimated there could be a trillion microbial species, the vast majority belonging to the two oldest domains of the tree of life, the archaea and the bacteria. The study, by Jay Lennon and Kenneth Locey of Indiana University, used microbial, plant and animal datasets representing more than 5.6 million microscopic and non-microscopic species from 35,000 locations across all the world’s oceans and continents. Lennon explained that “until recently, we’ve lacked the tools to truly estimate the number of microbial species in the natural environment. The advent of new genetic sequencing technology provides a large pool of new information. Microbial biodiversity, it appears, is greater than we ever imagined.”
But it’s probably not quite correct or completely useful to “measure” biological importance by—or at least only by—number of species. One problem is that the concept of a species was invented for describing multicellular organisms; the same concept doesn’t quite work for bacteria. What about using population size—the number of individuals—as a measure of ecological importance? In a paper from 1998 titled “Prokaryotes: The Unseen Majority,” William Whitman and colleagues estimated that the total number of bacteria and archaea are of the order of magnitude of ten to the thirtieth power—that’s a one with thirty zeros after it, or more than a billion-billion billion. In other words, a lot. Vastly more than any other kind of organisms.
Or what about comparing the biomass—the living weight—of multicellular and single-celled organisms to estimate their ecological importance? Whitman and his coauthors estimated the total biomass of all prokaryotes—the bacteria and archaea—on Earth to be about the same as that of all multicellular organisms. They also wrote that prokaryotes contain the largest pool of nitrogen and phosphorus—essential ingredients of life—in all living organisms. This vast “unseen majority” of microbes are the equals of the multicellular minority in biomass. And that’s a good thing. Bacteria and archaea are founding members of the biosphere, and without them, it wouldn’t function as it does, and we wouldn’t be here. Although a few of them make us sick, we owe our lives to them.
But in describing Earth as the “Planet of Bacteria,” Gould was really only half right. Where there are bacteria, there are viruses. Are, and probably always have been. Before we can make any sense of the world of viruses, we need a quick refresher course. What are viruses, anyway? Are they alive?
First, let’s just remember the fundamental law of physics called the “second law of thermodynamics” or “law of entropy.” It simply states that concentrated energy always dissipates; it flows from hotter to colder until everything is the same temperature. A corollary is that things spontaneously get more disorganized, more random, unless energy is added from somewhere else and work is done to reorganize and reorder them. You know all about this law from firsthand experience. Dirty dishes don’t do themselves. Something dropped doesn’t pick itself up off the floor.
But something called “information” can create a ripple in the downhill flow of energy in the universe, like a rock in a river can create a wave where water—briefly—flows uphill. Living things figured out how to use that trick sometime around 4 billion years ago here on Earth. Using information-containing molecules of RNA or DNA, living things managed to tap the flow of solar energy and chemical energy on the planet to organize little packets of order in the entropic universe. “Energy is the only life,” wrote William Blake, the English mystic poet. “Energy is Eternal Delight.”
But… so… what is life? Although the definition remains somewhat fuzzy, you would probably get most biologists to agree that living things have cells in which genetic information produces processes that harvest energy to maintain their orderly structure and reproduce themselves. The genetic information, stored mainly in DNA-containing “genes,” controls the assembly of proteins that make all the rest of the battle against entropy happen. Bacteria can do this with a few thousand genes; multicellular organisms usually have a few tens of thousands of genes.
Viruses don’t fit neatly into this picture. These little assemblages of up to a few hundred genes and some protein components don’t have cells and can’t reproduce themselves. They are sometimes called “the ultimate parasite,” and described as “hijacking” or “commandeering” or “subverting” the cellular machinery (of both prokaryotes and eukaryotes) to reproduce themselves. That value-loaded terminology portrays them as if they somehow are cheating, or that it is a negative thing to be able to do that to nice, orderly organisms. Flying their skull-and-crossbones flags, the virus ships swoop in to steal the gold and silver looted from… No, wait a minute. That analogy doesn’t work… British pirates were taking the gold and silver the Spanish had stolen from the Aztecs and Incas; it didn’t belong to the Spanish in the first place. Whose energy is it in those cells that viruses are “pirating.” We could just as easily think of viruses as “outsmarting” or “outmaneuvering” their host cells. Why waste a lot of time and energy making your own cells, a virus might be thinking, if you can get someone else’s cells to make copies of your genetic information and release multi-billions of copies of it? The virus “lifestyle” has been a very successful strategy, whether you call it “life” or not.

The quest to capture energy with information has created what has been called a “coevolutionary arms race,” as organisms compete to tap the finite energy flows of Earth. That arms race between prokaryotes and viruses has undoubtedly been going on since the beginning of life. Once multicellular organisms arose, they got into the fray too. There are no “winners” yet, and none in sight, of course. The key to understanding why this appears to be an endless race is the term “coevolutionary.” When a mutation creates a viral variant that is able to successfully attack a bacterium, natural selection within the bacterial population “fights back” by increasing the proportion of genetic variants that aren’t susceptible to the viral attack. But then further mutations in the virus create another evolutionary cycle, generating more diversity. A study by Chi Xue and Nigel Goldenfeld of the University of Illinois published in 2017 explained how coevolution could maintain diversity, as a summary in ScienceDaily described:
“There is remarkable biodiversity in all but the most extreme ecosystems on Earth. When many species are competing for the same finite resource, a theory called competitive exclusion suggests one species will outperform the others and drive them to extinction, limiting biodiversity. But this isn’t what we observe in nature. Theoretical models of population dynamics have not presented a fully satisfactory explanation for what has come to be known as the diversity paradox. Using bacteria and their host-specific viruses as an example, the researchers showed that as the bacteria evolve defenses against the virus, the virus population also evolves to combat the bacteria. This “arms race” leads to a diverse population of both. This coevolutionary arms race is sufficient to yield a possible solution to the diversity paradox.”
What about what has been called “virodiversity”? How many species of viruses might there be? A big problem in answering this question is that viruses don’t fit into the biological species concept very well, not even being able to reproduce themselves. The formal description and classification of viruses is the responsibility of the International Committee on Taxonomy of Viruses (ICTV). Historically, virus taxonomy has been based on their shape, whether they use DNA or RNA, their mode of replication, their hosts, and the types of disease they cause. The 2019 ICTV Master Species List includes 5,560 named virus “species”—fewer than the known species of mammals. It’s great fun to browse this list if you have a poetic ear. Consider just a few named viruses: raspberry bushy dwarf virus, tobacco albetovirus, white spot syndrome virus, saccharomyces 20S RNA narnavirus, Ourmia melon virus, melon aphid-borne yellows virus, Ebola virus, Marburg virus, falcon aviadenovirus A, beak and feather disease virus.
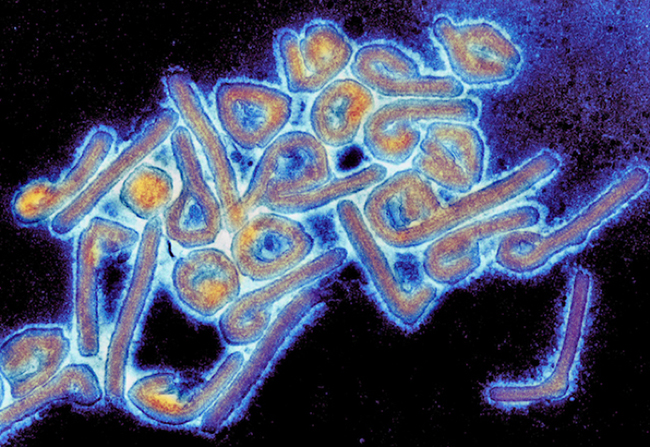
Modern techniques for sequencing genetic material from environmental samples “have revealed a staggeringly large virome everywhere in the biosphere,” according to a 2017 article titled “Virus Taxonomy in the Age of Metagenomics” written by a group of leading virologists led by Peter Simmonds. “Metagenomic data are changing our views on virus diversity and are therefore challenging the way in which we recognize and classify viruses,” they wrote. Far more viruses are now known to exist from genetic sequence data than have been described and classified by the ICTV. These scientists cite the case of a virus family called Genomoviridae, which currently includes only a single classified virus, although more than 120 possible members have been identified from genetic sequences collected from a wide range of environments. The ecology of these unclassified viruses—their hosts, habitats, mode of transmission, and pathogenesis—are currently unknown.
_______
So far, we’ve seen that there is a whole lot of diversity in the biosphere, especially in life’s ancient lineages, the archaea and bacteria, and the viruses that have coevolved with them. We briefly touched on the idea that competition for energy among life forms generates diversity through a coevolutionary arms race, in which the attacker and the attacked continually evolve genetic tools to outsmart each other. Such a coevolutionary arms race may explain the diversity of bacteria and viruses.
Evidence for the coevolution of bacteria and viruses is found in unusual sequences of DNA in the genomes of bacteria, called CRISPRs—an abbreviation for “clustered regularly interspaced short palindromic repeats.” Palindromes are words or phrases whose letters are the same backwards and forwards, like “kayak” or “Murder for a jar of red rum.” Prokaryotic genomes contain palindromic sequences of the four base-letters of DNA, A, C, T, and G, such as GTTCCTGTA-ATGTCCTTG. These CRISPR sequences represent a record of past viral infections; they act as spacers between sequences of DNA from viruses that previously infected the bacterium, and function to detect and destroy DNA from similar viruses. They are a kind of bacterial immunity mechanism or antiviral defense system. Understanding of CRISPRs and their function has been used to develop diagnostic tests for diseases and for gene “editing” and genetic “engineering.”
Coevolutionary struggles between humans and both viruses and bacteria have also left their genetic “fossils” in our genomes. Kelly Pittman and coauthors gave examples of the genetic responses to infectious diseases in a 2016 article in the journal PLOS Pathogens titled “The Legacy of Past Pandemics: Common Human Mutations That Protect Against Infectious Disease.” They conclude by bringing up the coevolutionary theme again:
“For millennia, pathogens and human hosts have engaged in a perpetual struggle for supremacy. From the earliest recorded smallpox epidemics around 1350 B.C.E. to the Black Death due to Yersinia pestis in the Middle Ages and continuing to modern times with HIV, there has been a continuous clash between pathogens and human hosts. But past pandemics are more than just ancient history—they are drivers of human genetic diversity and natural selection. Pathogens can dramatically decrease survival and reproductive potential, leading to selection for resistance alleles and elimination of susceptibility alleles. Studies identifying and characterizing alleles associated with infectious diseases from around the world have led to a better understanding of how the history of past pandemics is written in our genomes. The selective force of infectious diseases has had lasting impacts on our genetic susceptibility to ancient and emerging infections as well as autoimmune and chronic diseases. This story is ongoing and changes will continue to be written into our genomes by new and future infectious diseases.”
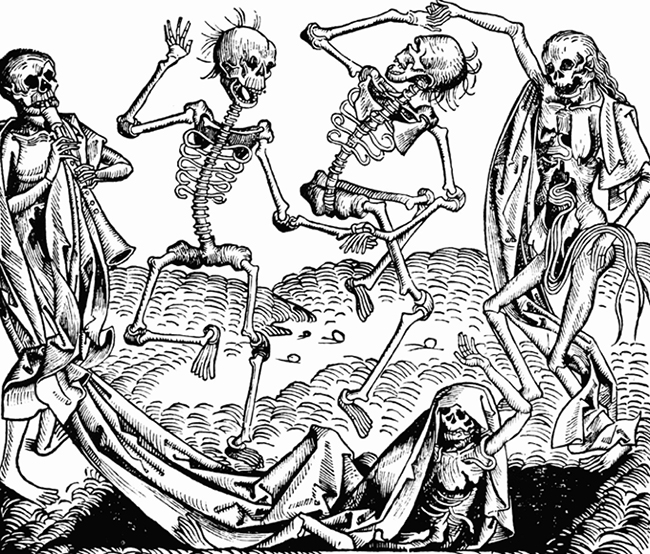
With all of this coevolution going on among viruses, prokaryotes, and multicellular organisms over millions and billions of years, it shouldn’t really come as a surprise that some genes have been jumping around and are shared among these groups. Viral infections are one mechanism for moving genes across branches of the phylogenetic tree of life, a process called horizontal gene transfer. A 2017 paper by Shahana Malik and colleagues, published in the journal Frontiers in Microbiology, explains how viruses can share their genes with a variety of cellular organisms that are not their hosts, and seed new genetic diversity across the major branches of the tree of life. “Organisms can form partnerships with other organisms and live in communities. For example, many bacterial and archaeal species reside in and on the human body and constitute the human microbiota,” the authors wrote. “Viruses that infect archaea and bacteria, for example, are not known to infect eukarya. However, they may still interact in nonharmful ways with organisms they do not infect.”
Let’s take a closer look at the living microbiome and virome of our bodies. Each of us is estimated to contain about 37 trillion human cells, and at least that many (some estimates are three times more) bacterial cells, according to David Quammen in his 2018 book The Tangled Tree: A Radical New History of Life. There are thought to be at least 1,000 kinds of bacteria in the human intestinal tract, and maybe ten times that many in and on our whole body. And it has been estimated that there are ten times more viruses in and on us than bacteria. Most of the bacteria and viruses are not pathogenic, obviously, but the ecology and evolution of our microbiome and virome is still mostly mysterious. Most of the viruses are the kind that attack bacteria, called bacteriophages. The “good” bacteria in our guts and on our skin tune our immune systems and help fight off attacks by bacterial pathogens and parasites. These bacteria may also provide a line of defense against harmful viruses.
Our history of evolutionary interaction with viruses over time has left a legacy of virus genes in the human genome. “What Percent Virus are You?” asks the title of an article summarizing the state of knowledge about this subject in 2016. The answer, based on comparing DNA sequences in humans with those of viruses, is that about 8 percent of our genes originally came from viruses. When first incorporated into human genes, viral gene sequences might have disrupted the function of those genes, perhaps causing latent disease or cancer. But in some cases, coevolution apparently reduced the negative effects, gradually “domesticating” the viral DNA. And sometimes that DNA apparently turned out to produce proteins or have other effects that were adaptive and beneficial to its carriers, and the viral genes were retained.
In fact, research suggests that multicellular organisms like ourselves wouldn’t exist without genes borrowed from viruses. Molecules that allow cells to fuse, as they do in sexual reproduction and when forming tissues and organs, seem to be derived from viral genes. Bits of viral DNA incorporated into our cells—called “endogenous retroviruses”—have been found to have diverse benefits, from increasing the ability of our human ancestors to digest starch to priming our immune systems to fight off diseases. Although viruses are most often studied as pathogens, more and more cases of viruses that are beneficial to their hosts are being discovered. They have been found in bacteria, insects, plants, animals, and fungi. How these beneficial interactions, which have been called “viral mutualistic symbioses,” evolve is still poorly understood.
“Everywhere you look, viruses seem to be playing a crucial role in evolution,” says Luis Villarreal, director of the Center for Virus Research at the University of California, Irvine. “I would argue that they are the most creative genetic entities that we know of.” Eugene Koonin, of the National Institutes of Health, proposed in a 2016 article that “host–parasite coevolution appears to be one of the key driving factors of evolutionary transitions,” and that new genetic combinations created in the coevolutionary process between hosts and genetic parasites “leads to increased organizational complexity of biological systems.”
_______
Paul Sharp, in a paper titled “Origins of Human Virus Diversity” published in 2002, wrote that
“The diverse consequences of different viral infections reflect both the history and the nature of their ongoing evolution. Members of at least 20 of these families infect humans. Human viruses … evolve in diverse ways and at rates differing by up to six orders of magnitude. Also, different viruses have been transmitted to humans from other species at various stages of our history. The term “emerging viruses” was coined primarily to cover those that appeared within the last century. Among these, some have spread epidemically in their new host, while others cause recurrent outbreaks but have not become established in the human population. Other viruses emerged thousands of years ago and have stayed. Some may have been introduced only once, whereas others are intermittently reintroduced from their natural reservoir. Finally, some viruses may not have been acquired by crossspecies transmission at all, but rather have always been with us, i.e., they infected our ancestors even before they had evolved to become humans.”
In 2012, a paper titled “Human Viruses: Discovery and Emergence,” Mark Woolhouse and colleagues reported that there were then 219 virus species known to be able to infect humans, with three or four new ones being reported each year (there must be 250 or more known by now). Not all of these are pathogenic, or at least not severely pathogenic. Throughout our evolution, we humans have been exposed to a vast reservoir of viruses. Viruses are very genetically diverse, and new genotypes, strains, and species can evolve very rapidly because of their mode of reproduction, over periods of months, years, and decades. More than two-thirds of human viruses are shared with other mammals and birds, and are capable of cross-species transmission. The emergence of novel human viruses into the human population is a natural and ongoing biological process. It is often driven by how people interact with the ecosystems around them, especially with birds and mammals. Woolhouse and his coauthors argued that “a better understanding of the emergence of new human viruses as a biological and ecological process will allow us to refine our currently very crude notions of the kinds of pathogens, or the kinds of circumstances, we should be most concerned about, and so direct our efforts at detection and prevention more efficiently.”
We know that lots of viruses are lurking out there in ecosystems, just waiting for their chance to jump to other species, including ours. But we have no clear idea of how many there are. Some virologists have proposed that we’d be less surprised and better prepared to respond if we had a catalogue of all of those zoonotic viruses waiting to make the jump. In a paper titled “A Strategy to Estimate Unknown Viral Diversity in Mammals” published in 2013, Simon Anthony and numerous coauthors tried to identify all of the viruses infecting one mammal species, a bat called the Indian Flying Fox, Pteropus giganteus. Using genetic sequencing techniques, they found 55 different viruses in this bat species. Assuming that every mammal species would host approximately this level of unique virus diversity, they then estimated that there are at least 320,000 different mammalian viruses. That’s a lot, but these researchers estimated that all of these viruses could be identified by a 10-year research effort costing $6.3 billion. That cost would be “a small fraction of the cost of many pandemic zoonoses,” they wrote. It was a painfully prescient statement, made before the global economy was smashed by the novel coronavirus.

The novel coronavirus now ravaging the world is officially named “severe acute respiratory syndrome coronavirus 2” —SARS-CoV-2 for short—by the International Committee on Taxonomy of Viruses. SARS-CoV-2 virus was originally transmitted from a mammal to humans, a process known as zoonosis. It seemed to take the world by surprise, but really shouldn’t have—virus experts have been issuing clear warnings that this could and would happen again since the first SARS coronavirus (SARS-CoV-1) emerged into the human population in China in late 2002. That virus spread slowly around the world, often carried by airline travelers, eventually infecting more than 8,000 people in 37 countries, and killing about 10 percent of those infected. Not nearly as transmissible as the current novel coronavirus, by 2004 it had been controlled through quarantine measures, and it has never reemerged. That episode scared many scientists, who warned that it could happen again anytime, and a scientific search began to understand the ecology and evolution of SARS-related coronaviruses.
The capstone research paper on that topic, titled “Discovery of a Rich Gene Pool of Bat SARS-Related Coronaviruses Provides New Insights into the Origin of SARS Coronavirus,” was finally published in 2017 by a large team from the Wuhan Institute of Virology led by Zheng-li Shi. Peter Daszak of the New York-based EcoHealth Alliance was the only non-Chinese coauthor.

The team from the Wuhan Institute of Virology sampled for coronaviruses in a single cave in Yunnan province. With biohazard-containment equipment and protocols, they looked for viruses in bat feces and anal swabs, and used genetic probes to detect coronaviruses. They found that the cave was a stewpot where coronaviruses containing all the genetic pieces of SARS-CoV-1 were circulating among four species of horseshoe bats roosting together there. This kind of situation, whether in this particular cave or another similar one, created the potential for genetic recombination of these viral components and probably cooked up SARS-CoV-1. Most of the samples that were positive for SARS-related coronaviruses were from Rhinolophus sinicus, the Chinese rufous horseshoe bat, but coronaviruses were also found in Rhinolophus affinis (intermediate horseshoe bat), Rhinolophus ferrumequinum (greater horseshoe bat), and Aselliscus stoliczkanus (Stoliczka’s trident bat). The Wuhan researchers concluded, based on analysis of genetic sequences of the new SARS-related coronaviruses they identified, that the direct ancestor of SARS-CoV-1 may have arisen from sequential recombination events between these bat coronaviruses prior to spillover to an intermediate host. They found bat SARS-related coronavirus strains with proteins that can all use the same receptor as SARS-CoV-1 to gain entry to human cells, suggesting that diverse SARS-related coronaviruses “capable of direct transmission to humans are circulating in bats in this cave. Our current study therefore offers a clearer picture on the evolutionary origin of SARS-CoV and highlights the risk of future emergence of SARS-like diseases.”
So, fifteen years after the 2002-2004 SARS scare, scientists finally figured out how the disease emerged into humans, and concluded their report with a warning: “This work provides new insights into the origin and evolution of SARS-CoV and highlights the necessity of preparedness for future emergence of SARS-like diseases.” Two years later, SARS-CoV-2 emerged—without the necessary preparedness—and here we are now.

There are around 1,200 species of bats, Order Chiroptera; bats make up about 20% of all mammal species. Bats are ecologically diverse and evolutionarily successful. Because they are highly mobile, often roost in large social groups, and are relatively long-lived, they are natural reservoirs of many viral pathogens, such as henipaviruses, rabies viruses, and SARS-related coronaviruses. Recent research on coronaviruses and henipaviruses shows that bats host these viruses on several continents, so it is probably a worldwide phenomenon.
The Wuhan researchers who untangled the probable story of how SARS-CoV-1 emerged noted that the cave in which they found coronaviruses simmering among mixed-species bat colonies was only half a mile from the nearest village. Although the coronavirus strains appeared to be capable of direct attack on human cells, an “intermediate host” was suspected. The prime candidate for the intermediate host in that outbreak is the masked palm civet (Paguma larvata), a cat-like wild carnivore that is eaten in China. Palm civets sold in live animal markets in Guangdong, China, were shown to be infected by the same strain of SARS-CoV-1 coronavirus as workers in those markets and staff at nearby restaurants serving civet meat. The civets are thought to have acquired the virus from bats. Kwok-Yung Yuen, a virologist at the University of Hong Kong who co-discovered the SARS-CoV-1 virus, gave a gentle warning that “we should not disturb wildlife habitats and never put wild animals into markets. Respecting nature is the way to stay away from the harm of emerging infections.”
We didn’t act with urgency on the warning from Chinese and other virologists after the first SARS outbreak. It took fifteen years of research to piece together the ecological and evolutionary story of how it emerged. But scientific research on the novel coronavirus has been rapid, and there already seems to be an emerging picture of where it came from and how it jumped to humans.
In a letter published in March 2020 in the journal Nature Medicine titled “The Proximal Origin of SARS-CoV-2,” Kristian Andersen and coauthors offered two hypotheses for its origin: natural selection in an animal host before zoonotic transfer, and natural selection in humans following zoonotic transfer. They noted that a coronavirus sampled from a horseshoe bat, Rhinolophus affinis, is genetically about 96 percent identical to SARS-CoV-2, but that the receptor-binding domain of its spike protein, with which it attaches to human cells, is not optimal for attacking them. On the other hand, they found that Malayan pangolins (Manis javanica), which are illegally imported into China from Vietnam and other nearby countries for food and medicinal purposes, carry coronaviruses with receptor-binding domains that are strongly similar to SARS-CoV-2. This evidence seems to point to a jump from bats to an intermediate host, perhaps pangolins or another of the many other species of wild mammals sold for food in the Wuhan market, and then to humans. The final evolutionary touches that make this novel coronavirus so transmissible may have occurred through adaptation during undetected human-to-human transmission, the authors speculate. That final genetic tweaking of the virus may have allowed the pandemic to erupt.

Eating meat from wild birds and mammals are not the only risks for zoonotic transmission of new pathogens from animals to humans. People everywhere surround themselves with the domestic animals they raise and eat. Anyone who works in close proximity to poultry and pigs are at increased risk for zoonotic transmission of strains of influenza virus that we share with these animals. In 2016, a novel coronavirus carried by bats infected pigs in Guandong province. Called swine acute diarrhoea syndrome coronavirus (SADS-CoV), it killed tens of thousands of piglets before the epidemic abated. A related coronavirus already circulates in humans, generally causing mild common-cold symptoms. A large team of authors from—guess where? —the Wuhan Institute of Virology analyzed this outbreak and concluded with a chilling warning in a 2018 paper published in the journal Nature: “We found that there were striking similarities between the SADS and SARS outbreaks in geographical, temporal, ecological and aetiological settings. This study highlights the importance of identifying coronavirus diversity and distribution in bats to mitigate future outbreaks that could threaten livestock, public health and economic growth.”
_______
Humans became masters of manipulating nature because our genetic evolution made us into a big-brained, cultural species. In the competition for energy that drives all life, our ancestors learned how to harness fire, domesticate other plant and animal species, and even tap the fossil energy of ancient ecosystems in the form of coal, oil, and natural gas. The idea of human control of nature has been around for at least a few centuries, but a major turning point in this mythology happened around the time of World War II. In 1939, a Swiss chemist named Paul Müller discovered that a synthetic organic compound called dichlorodiphenyltrichloroethane—DDT for short—killed many insects on contact. DDT was used in the war to control mosquitoes that carried malaria and body lice that carried typhus, saving the lives of many troops and civilians. Müller was awarded the Nobel Prize for his discovery in 1948, and DDT use became widespread, promoted by chemical companies and government agencies. At around the same time, in 1942, the antibiotic penicillin was first purified and used by the Allied military during the war. It became more widely available at the end of the war, and its discoverers were awarded the Nobel Prize in Medicine in 1945.
It seemed that humans had taken a giant leap toward the control of nature, especially of the infections and diseases that had plagued us forever. But the control of nature would prove, once again, to be an impossible dream. By the early 1950s, mosquitoes in some parts of the world had evolved resistance to DDT. By the early 1980s, about 450 species of insects were resistant to all of the classes of insecticides used at the time. Penicillin-resistant Staphylococcus aureus bacteria were found by 1947, only a few years after the widespread use of the antibiotic began. By now, some strains of Staphylococcus have evolved resistance to almost every antibiotic known, including methicillin, a drug of last resort. Ah, the coevolutionary arms race again! Adios, dreams of the control of nature.
“He’s too big for his britches,” my dad used to say about someone full of his own importance, conceited, overconfident and closed-minded, bound to cause harm to himself or others because of his attitude. The phrase was apparently first used by the American frontiersman Davy Crockett, in a narrative of his adventures published in 1835, referring to Andrew Jackson, under whom Crockett had served and who went on to become probably the most divisive president in U.S. history until the current one. It could apply to our own species; we have an attitude problem, we’re too big for our britches. Fortunately, fate gives us a chance, time and time again, to learn and change. This is one of those times.
The Merriam-Webster dictionary says that “English picked up both the concept of hubris and the term for that particular brand of cockiness from the ancient Greeks, who considered hubris a dangerous character flaw capable of provoking the wrath of the gods. In classical Greek tragedy, hubris was often a fatal shortcoming that brought about the fall of the tragic hero. Typically, overconfidence led the hero to attempt to overstep the boundaries of human limitations and assume a godlike status, and the gods inevitably humbled the offender with a sharp reminder of his or her mortality.”
Rachel Carson’s original title for her last and most influential book was The Control of Nature. Her literary agent suggested instead that she take the evocative title of one of her chapters for the whole book, and call it Silent Spring. “Carson asked the hard questions about whether and why humans had the right to control nature,” says her biographer Linda Lear.
“She wrote with passion and employed military metaphors to illustrate that ‘we are engaged in a war against nature that will inevitably destroy us as well as our supposed enemies,’” wrote Brian Payton in a 2002 biographical sketch of Carson published by the NASA Earth Observatory. In a chapter of Silent Spring titled “Nature Fights Back,” Carson describes the dynamic, homeostatic character of intact ecosystems—sometimes called “the balance of nature”—in which a natural web of ecological interactions generally regulates the populations of pest species. When humans try to kill a “target” insect with an insecticide, Carson explained, that often punches a hole in that homeostatic web, leading to far more pest problems than existed before. Carson wrote that “the ‘control of nature’ is a phrase conceived in arrogance, born of the Neanderthal age of biology and philosophy when it was supposed that nature exists for the convenience of man.”
Carson was right to name both biological and philosophical dimensions of the problem of “the control of nature.” Biologically, she knew that in an ecologically interconnected and interdependent world, one species can’t “win” an ecological war. If you spray mosquitoes with DDT, pretty soon some evolve resistance to DDT. If you blast a bacterial disease with antibiotics again and again, pretty soon some germs evolve resistance to antibiotics. If you vaccinate against a virus, pretty soon the virus mutates and does an end run around the immunity the vaccine created. There is no way to “control nature” or win a “war against nature.” We are part of nature, and what we do to it, we do to ourselves. As Walt Kelly had Pogo say, “We have met the enemy and he is us.”

Ironically, the threat from emerging diseases is partly the result of our success in the “war” against such diseases. Antibiotics that bring control over bacterial attacks on our bodies like pneumonia and strep and vaccines that partially protect humans from the scourges of measles, smallpox, polio, and cholera are in significant measure responsible for the “population explosion”—the rapid, exponential increase in the Earth’s human population that begin just after World War II. The human population was less than 3 billion in 1950, but by 2020 has climbed to 7.8 billion people. The United Nations projects that the world’s human population will reach a maximum of 10.9 billion at the end of this century. The sharp steepening of the population-growth curve is due to the time lag between lower death rates brought about by our antibiotics and vaccines and lower birth rates caused by social, cultural, and economic changes, including the availability of modern family planning methods. Human overpopulation is a serious ecological problem. Our sheer numbers lead to a level of energy and resource use that is changing the dynamics of the biosphere, Earth’s life support system, in unpredictable ways. What epidemiologists can predict is that dense human populations (urbanization is accelerating everywhere) are perfect media for emerging pandemic diseases. Our cities are petri dishes for their spread.

_______
J.B.S. Haldane, Stephen Jay Gould, and Rachel Carson challenged us to rethink our place in nature and the meaning and purpose of human life. And now SARS-CoV-2 challenges us again. We can reach for help in a few more places…

The Gaia hypothesis was a thought-provoking and controversial concept developed in the early 1970s by James E. Lovelock, an eclectic British scientist with a Ph.D. in medicine and interests in atmospheric chemistry, and Lynn Margulis, an American evolutionary biologist. Their joint 1974 paper proposing the idea was titled “Atmospheric Homeostasis By and For the Biosphere: The Gaia Hypothesis.” Named after Gaia, the goddess of Earth and mother of all life in Greek mythology, the hypothesis proposes that living things interact with the physical components of the planet—the atmosphere, lithosphere, and hydrosphere—to form a complex, self-regulating, homeostatic, synergistic, symbiotic system. “Early after life began,” they argued, “it acquired control of the planetary environment and … this homeostasis by and for the biosphere has persisted ever since.” Drawing ideas from systems science at all scales, Gaia was sometimes portrayed as a single, living organism of planetary scale. The idea was provocative and compelling, but drew criticism from evolutionary biologists because in some formulations it seemed teleological—that is, goal-directed, purposive, and perhaps even conscious. Evolutionary theory since Darwin had rejected teleology as an explanation for biological structures and functions, instead viewing natural selection as the creative force in evolution.
While Lovelock brought the atmosphere and other physical systems into the picture at the planetary scale, Margulis brought symbiosis into the system at the smallest scales. Margulis’s work links back to our earlier discussion of bacteria and multicellular organisms. Her work, controversial at the time, revolutionized scientific understanding of the evolution of eukaryotes. She proposed that the internal “organelles” found in eukaryotic cells, such as the energy-processing mitochondria and photosynthesizing chloroplasts, were once independent bacteria that had been taken inside the larger, walled cells as mutualistic “endosymbionts,” where they provided new and powerful functions to those cells. Her hypothesis was at first ridiculed, but within a decade, genetic analysis of the genes in those mitochondria and chloroplasts proved that they were indeed prokaryotic in origin.
The Gaia hypothesis crept into The Hot Zone, a nonfiction book by Richard Preston published in 1994. The book described the true story of a novel filovirus, now called Reston virus, that showed up in a captive primate facility in Reston, Virginia, in 1989 among crab-eating macaque monkeys imported from the Philippines for medical research. Luckily, this virus—related to the extremely deadly Ebola and Marburg viruses from Africa—proved to be benign in humans, although deadly to the monkeys. The epizootic event was controlled. At the end of the book, Preston wrote that
“The emerging viruses are surfacing from ecologically damaged parts of the earth. Many of them come from the tattered edges of tropical rain forest … The tropical rain forests are the deep reservoirs of life on the planet, containing most of the world’s plant and animal species. The rain forests are also its largest reservoirs of viruses, since all living things carry viruses. When viruses come out of an ecosystem; they tend to spread in waves through the human population, like echoes from the dying biosphere.
In a sense, the earth is mounting an immune response against the human species. It is beginning to react to the human parasite, the flooding infection of people, the dead spots of concrete all over the planet, the cancerous rot-outs in Europe, Japan, and the United States, thick with replicating primates, the colonies enlarging and spreading and threatening to shock the biosphere with mass extinctions. Perhaps the biosphere does not “like” the idea of five billion humans.
Nature has interesting ways of balancing itself. The rain forest has its own defenses. The earth’s immune system, so to speak, has recognized the presence of the human species and is starting to kick in. The earth is attempting to rid itself of an infection by the human parasite.”
Interesting thoughts, but Preston’s description falls into the trap of teleological thinking for which the Gaia hypothesis was criticized earlier. For another, more equanimous analogy than that of a pissed-off Earth goddess, we can turn to the metaphor of the jeweled Net of Indra as described in the Avatamsaka Sutra, an ancient Buddhist text. Indra’s net is pictured as stretching infinitely in all directions. At each of the knots of the net is a glittering jewel; each jewel reflects all the other jewels and is itself reflected in all. This metaphor describes what was called “dependent co-arising,” paticca samupadda in Pali (the original language of the Buddhist canon). Robert Aitken, a modern Zen teacher, called it “the harmony of universal symbiosis.” I imagine Lovelock and Margulis wouldn’t mind that description of Gaia.
Coronavirus is giving us an opportunity, once again, to reconsider our anthropocentrism and human hubris. To get our bearings again in the time of coronavirus we need to “realize where we are and the infinite extent of our relations,” as Henry David Thoreau wrote in Walden. We are part of a coevolved and continually coevolving biosphere. From the viral DNA deep in our genes to the ancient endosymbiotic bacteria that power our cells, from the signatures of past pandemics we carry in our chromosomes to the microbiomes and viromes inside our bodies, from the diversity of species we live among and eat to the atmosphere we interpenetrate with every breath, we live in a world of dependent co-arising. Like it or not, we live within the harmony of universal symbiosis—coronavirus and all.
Sources and related links
- “Why are there so many freaking beetles? Science may finally have the answer.” Rachel Feltman, March 18, 2015. Washington Post.
- Beautiful Bugs: Beetle Collection Karlsruhe Museum of Natural History, Germany. Photo by Scott Lewis.
- Gould, 1996. Planet of the Bacteria. November 13, 1996. Washington Post.
- “Researchers find that Earth may be home to 1 trillion species.” May 2, 2016, National Science Foundation News Release.
- Whitman, William B., David C. Coleman, and William J. Wiebe. 1998. “Prokaryotes: The Unseen Majority. PNAS 95 (12): 6578-6583.
- Energy is Eternal Delight, William Blake etching from The Marriage of Heaven and Hell, 1790 (image courtesy of The William Blake Archive).
- “A virus-bacteria coevolutionary ‘arms race’ solves diversity by ‘killing the winner.’” January 2, 2018. ScienceDaily.
- International Committee on the Taxonomy of Viruses. ICTV Master Species List 2019 v2.
- Marburg virus (image courtesy of The University of Texas Medical Branch at Galveston).
- Pittman, et al. 2016. “The Legacy of Past Pandemics: Common Human Mutations That Protect against Infectious Disease.” PLOS Pathogens.
- Malik, et al. 2017. “Do Viruses Exchange Genes across Superkingdoms of Life?” Frontiers in Microbiology.
- Quammen, David. 2018. The Tangled Tree: A Radical New History of Life. New York: Simon & and Schuster.
- “What Percent Virus Are You?” Hannah Moots, December 14, 2016. FiveThirtyEight.
- “Viruses: The Unsung Heroes of Evolution.“ Garry Hamilton. August 27, 2008. NewScientist.
- “Our Complicated Relationship with Viruses.” ScienceDaily, November 28, 2016.
- Roossinck, Marilyn J. 2011. “The Good Viruses: Viral Mutualistic Symbioses. Nature Reviews Microbiology 9, 99–108.
- Koonin, Eugene V. 2016. “Viruses and Mobile Elements as Drivers of Evolutionary Transitions.” Phil. Trans. R. Soc. B
- Coronavirus, 2020. David S. Goodsell, RCSB Protein Data Bank.
- Hu, et al., 2017. Discovery of a Rich Gene Pool of Bat SARS-related Coronaviruses Provides New Insights into the Origin of SARS Coronavirus. PLOS Pathogens.
- EcoHealth Alliance.
- Andersen, K.G., Rambaut, A., Lipkin, W.I. et al. 2020. “The Proximal Origin of SARS-CoV-2.” Nature Medicine 26, 450–452.
- Malayan pangolin (Manis javanica) (photo courtesy of Zaharil Dzulkafly/IUCN Pangolin Specialist Group).
- Zhou, et al. 2018. “Fatal Swine Acute Diarrhoea Syndrome Caused by an HKU2-Related Coronavirus of Bat Origin.” Nature.
- Carson, Rachel. 1962. Silent Spring. Boston: Houghton Mifflin. (Wikipedia entry)
- “The Control of Nature: Rachel Carson.” May 24, 2013.
- “The Life and Legacy of Rachel Carson.” (by Linda Lear).
- “Rachel Carson (1907-1964).” Brian Payton. 2002 Biographical sketch published by NASA Earth Observatory.
- The Triumph of Death (1562), by Pieter Bruegel the Elder. Museo del Prado, Madrid.
- “The Gaia Hypothesis.” (Wikipedia entry).
- James E. Lovelock and Lynn Margulis. 1974. “Atmospheric Homeostasis By and For the Biosphere: The Gaia Hypothesis.” Tellus 26 (1-2): 2-10.
- Preston, Richard. 1994. The Hot Zone: A Terrifying True Story. New York: Random House. (Wikipedia entry)
- Aitken, Robert. 1984. The Mind of Clover: Essays in Zen Buddhist Ethics. New York: North Point Press.
July 2, 2020 3:35 pm
Bruce,
An extraordinary piece of work! I can’t encourage you enough to get this published in a high profile magazine or journal. You carefully walk the reader through deep evolutionary earth, the complexity of microbiology, the history and lessons of ecology, and the dharma.
Am buzzing from having just read it …
… th
July 3, 2020 2:41 pm
Thank you for the positive feedback, Tim. Glad if the piece left you buzzing with ideas. I’ll certainly think about trying to publish it, or something similar, elsewhere. Bruce
July 6, 2020 1:35 pm
Bruce,
This essay has shed light on grave misinterpretations that have been dominating our media (including the viewpoints of many scientists and conspiracy theorists, unfortunately), since the onset of the current pandemic. In particular, arguing for a health-related menace of wildlife trade being a likely result of human attempts to control nature has not been easy – much the same way arguments on climate change tend to get clouded by side shows.
I have been facing a challenge authoritatively connecting the emergence of SARS-CoV-1 to the use of bats, palm civets, pangolins etc, leave alone how a similar link could have spiralled in the emergence of SARS-CoV-2.
Evans